Background
Natural gas as a fuel for internal combustion engines is an attractive alternative to diesel due to lower fuel cost and lower emissions resulting from naturally clean combustion. Natural gas engines are used for on-highway trucks, off-road vehicles, locomotives, marine vessels, and stationary applications. Since natural gas has a higher hydrogen-to-carbon ratio (approximately 3.8 H/C) compared to diesel (approximately 1.8 H/C), they emit approximately 25% less CO2 than diesel engines, while emissions of sulfur oxides, soot, and particles are virtually non-existent. CO2 is classified as a greenhouse gas (GHG) and is the primary focus with respect to mitigating climate change. Other GHGs include CH4 and N2O which have a much higher global warming potential than CO2, 28 and 265 times that of CO2, respectively. So, with respect to GHGs, the CO2 advantage of natural gas can disappear quickly as CH4 is emitted from the engines. The desire to reduce CH4 emissions from natural gas engines is particularly urgent at this time due to the worldwide focus on climate change and GHGs and is made more critical by the fact that an effective oxidation catalyst for CH4 does not exist. The objective of this qualitative study was to investigate the impact of piston design features on CH4 emissions, while maintaining piston reliability using top ring temperature as a gauge.
Approach
Three piston designs were analyzed using combustion CFD to estimate the impact of the top ring position and bowl design on the unburned hydrocarbon emissions. It is an established practice that reduced crevice volume (e.g., from raising the top ring) has a strong impact on reducing unburned HC. However, raising the top ring results in higher top ring temperatures which result in oil coking and other failures in operation. Therefore, these designs were also analyzed using thermal FEA to assess the impact of the design on piston temperatures. These analyses were coupled in that results from the respective analyses were used as boundary conditions in the other in an iterative fashion until stable results were obtained. The piston designs and thermal analysis results are shown in Figure 1. The baseline piston was a standard combustion bowl with a typical top ring placement. The second design was a standard bowl with a raised top ring to reduce the crevice volume. The third design had an inverted bowl which served to reduce the crevice volume by eliminating the bowl and moving the edge of the piston down toward the typical top ring location. In addition to differences in the bowl and top ring location, the oil gallery was also different between the designs which, while not ideal from a consistency standpoint, was necessary due to the differences in the designs. The oil gallery position and design have a direct impact on the piston crown and ring temperatures.
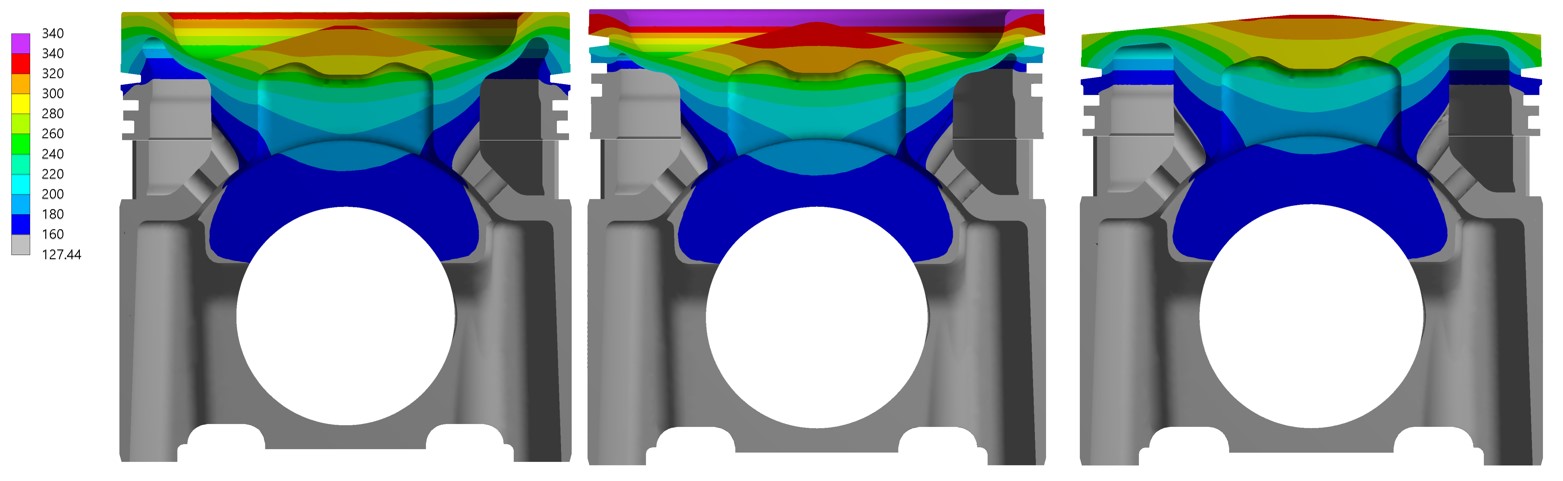
Figure 1: Piston temperature contours
Accomplishments
Combustion CFD was successfully used to estimate unburned hydrocarbon emissions as illustrated in Figure 2. The crevice volume was the main contributor to unburned HC for traditional combustion bowl designs. Raising the top ring to reduce the crevice volume was effective up to the point that the top ring temperature approached the design limit. Piston design features like the inverted bowl can decouple the relation between top ring position and top ring temperature; however, cooler piston surface temperatures lead to flame quenching and an additional source of unburned HC.
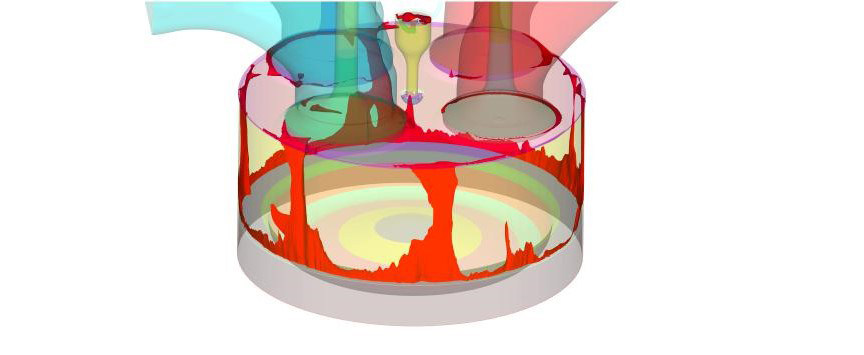
Figure 2: Contours of unburned hydrocarbons emitted from crevice volume.