Click below to jump to an article of interest.
FAST solutions to energy storage
The FAST Commissioning for Pumped Storage Hydropower (PSH) prize competition selected a Southwest Research Institute PSH concept among its four grand prize winners.
The FAST prize — which stands for “Furthering Advancements to Shorten Time” Commissioning for PSH — aims to reduce commissioning times of PSH projects by half, while reducing both cost and risk. The National Renewable Energy Laboratory (NREL) administers and executes these prizes on behalf of the U.S. Department of Energy’s Water Power Technologies Office.
Electric power systems use PSH for load balancing. The method uses the gravitational potential energy of water, pumped from a lower-elevation to a higher-elevation reservoir using low-cost, off-peak surplus electric power to run the pumps. During periods of high electrical demand, the stored water is returned to the lower reservoir, driving turbines to produce electric power. Although the losses from the pumping process mean it consumes more energy than it generates, the system creates value by providing more electricity during periods of peak demand, when electricity prices are highest.
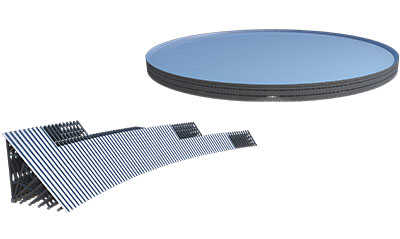
Courtesy of 2019 Google Map data
To accelerate pumped storage hydropower development, SwRI has developed a modern version of a 19th-century steel structural dam to impound water in a reservoir. The circular dam above is composed of modular sections that can be rapidly assembled using redesigned steel support frames made of standard W-beams and S-beams (left). The frame and water-tight faceplate modules are put in place using mobile heavy-lift cranes.
Selected from a field of 22 finalists, two SwRI concepts were among nine that moved on to the “incubation stage” of the competition. Following pitch presentations on October 8, SwRI was named one of the grand prize winners for its concept to lower the costs and construction time for building PSH reservoir dams.
“Our concept explores how to adapt a successful, but little-known 19th century steel dam construction technique to accelerate PSH development in the 21st century,” said Dr. Gordon Wittmeyer, a hydrologist in SwRI’s Chemistry and Chemical Engineering Division and the Institute lead for PSH. He is working with Dr. Biswajit Dasgupta, an SwRI structural engineer with significant experience in the hydropower industry, to develop a modular design concept for structural steel dams. The technique could cut dam construction costs by one-third and reduce construction schedules by half.
“It’s significant that the two SwRI concepts were both selected to move forward to the incubator stage of the competition,” said Eric Thompson, a program manager in SwRI’s Mechanical Engineering Division. He worked with SwRI’s Kevin Supak on the other concept. They analyzed the potential of using an array of shallow, interconnected reservoirs combined with packaged turbine units to reduce the PSH construction schedule, with the goal of bringing electric power to the grid sooner and improving the project return on investment and net value.
SwRI, UTSA Connect through research
The University of Texas at San Antonio and SwRI are collaborating to understand supercritical carbon dioxide (sCO2) energy cycles and metal degradation in 3D printed materials. The research projects are supported by $125,000 grants from the SwRI-UTSA Connecting through Research Partnerships (Connect) Program.
The program is sponsored by the Office of the Vice President for Research, Economic Development, and Knowledge Enterprise at UTSA and the Executive Office at SwRI. Connect grants enhance greater scientific collaboration between the two institutions and increase both UTSA’s and SwRI’s research funding with cross-campus programs.
Modeling sCO2 power cycles
One Connect grant is funding data acquisition to create a computational model for supercritical carbon dioxide (sCO2) energy cycles. The work is led by Dr. Jacob Delimont of SwRI’s Mechanical Engineering Division and Christopher Combs of UTSA’s College of Engineering.
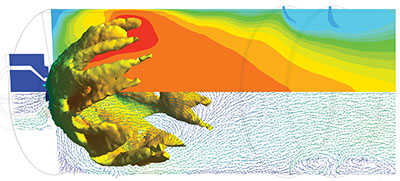
Through a Connect grant, SwRI and UTSA are collecting visual data of fuel burning in the sCO2 to validate a computational model for a direct-fired sCO2 combustor.
When carbon dioxide is held above a critical temperature and pressure, it reaches a supercritical state, acting like a gas while having the density of a liquid. The nontoxic, nonflammable sCO2 is a highly efficient fluid for generating power because small changes in temperature or pressure cause significant shifts in its density. Typically, current power plants use water as a thermal medium in power cycles. Replacing water with sCO2 increases efficiency by as much as 10 percent.
Because of the high fluid density in sCO2 power cycles, power plant turbomachinery can be one-tenth the size of conventional power plant components, providing the potential to shrink the environmental footprint as well as the construction cost of any new facilities.
Delimont and Combs plan to work with a direct-fired sCO2 cycle, which involves adding fuel and oxygen directly into the CO2 stream, causing it to combust, release heat and create sCO2. This new power cycle allows for higher efficiency and lower greenhouse gas emissions.
“This power cycle facilitates the capture of 100 percent of the CO2 emissions that would otherwise end up in our atmosphere,” Delimont said. “The captured CO2 has many potential uses, including several applications in the oil and gas industry and the carbonation in everyday soft drinks.”
Direct-fired sCO2 power generation is such a new technology that very little is known about the combustion process. Delimont and Combs will collect data to validate a computational model for an sCO2 combustor.
To visualize the burning of the sCO2 fuel, UTSA will supply optical lenses and laser systems as well as Combs’ expertise in the optical techniques needed to visualize the flame in the direct-fire combustor.
“Once we can visualize the combustion process, we can use computational models to design the necessary combustion equipment to make this power generation process a reality,” Delimont said.
Metal Degradation
W. Fassett Hickey of SwRI’s Mechanical Engineering Division and Brendy Rincon Troconis of UTSA’s College of Engineering are studying how susceptible additively manufactured materials are to hydrogen embrittlement. Additive manufacturing (AM) is an increasingly popular method of creating meticulously designed metallic parts through 3D printing. The method’s applications are practically limitless, but Hickey and Troconis are particularly interested in the performance of AM materials for aerospace and oil and gas applications.
Hydrogen sulfide (H2S) gas is commonly encountered during oil and natural gas production. When the atomic hydrogen in hydrogen sulfide is liberated, it can absorb into pipeline material and down-hole tools and degrade material performance through a phenomenon known as hydrogen embrittlement. The results can be devastating. For example, in 2014, the phenomenon caused large cracks in the pipelines at Kazakhstan’s largest oil field, causing a two-year shutdown for repairs.
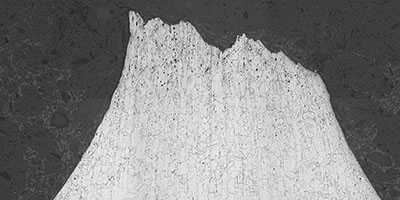
An SwRI-UTSA Connect grant is supporting hydrogen embrittlement research, looking at material degradation mechanisms at microstructural levels with the ultimate goal of designing additively manufactured parts that are less susceptible or even immune to these threats.
“Atomic hydrogen is an unintended alloying element that can wreak havoc on even the most advanced and modern alloy systems,” Hickey said.
The project will focus on the hydrogen embrittlement mechanisms in additively manufactured nickel-based alloy 718, with future goals to design AM parts that are less susceptible or even immune to these threats.
Hickey and Troconis are studying hydrogen embrittlement on a molecular level to understand how hydrogen atom location affects the integrity of the metal material. Unique SwRI facilities allow testing materials under the high pressures and elevated temperatures typical of drilling environments, including exposure to gaseous hydrogen up to 3,000 pounds per square inch and 500 degrees Fahrenheit. Using UTSA’s thermal desorption spectrometer and scanning Kelvin probe force microscope, the team will isolate the hydrogen-alloy interaction and spatially resolve where the hydrogen resides within the alloy microstructure.
“Additive manufacturing brings a lot of exciting new possibilities,” Hickey said. “We’re working with new designs that weren’t possible with traditional machining and fabrication methods. If we can better understand the underlying mechanisms of hydrogen embrittlement in AM materials, the fabrication parameters and post-processing parameters of AM parts can be designed to prevent hydrogen embrittlement. This would ultimately expand the possibilities and applications for these AM materials.”
Studying Binaries to Make Heads or Tails of Planet Formation
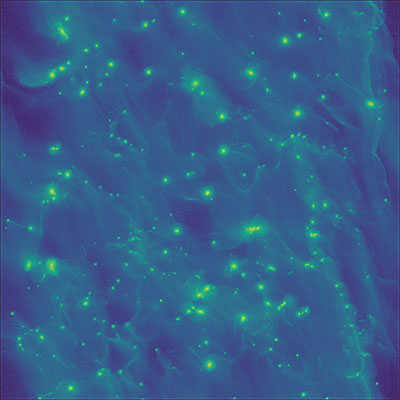
Courtesy of Steward Observatory/University of Arizona/Li/Youdin/HST/StSci/SwRI/Simon Porter
An SwRI-led team performed 3D simulations of the streaming instability model of planet formation, where particle clumping triggers gravitational collapse into planetesimals. This snapshot from the simulation shows the vertically integrated density of solids, projected on the protoplanetary disk plane.
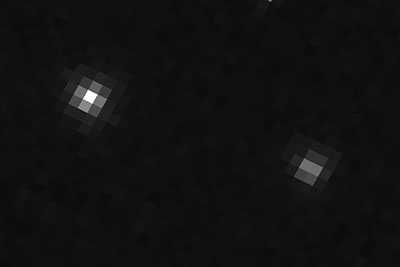
Courtesy of Steward Observatory/University of Arizona/Li/Youdin/HST/StSci/SwRI/Simon Porter
SwRI scientists used Hubble Space Telescope images of Kuiper Belt binaries to determine that 80% orbit in the same direction as the planets. This research helps scientists improve planet formation models. Kuiper Belt object 2006 CH69, imaged on Jan. 26, 2017, is shown here.
SwRI led a team studying the orientation of distant solar system bodies to bolster the “streaming instability” theory of planet formation.
“One of the least understood steps in planet growth is the formation of planetesimals, bodies more than a kilometer across, which are just large enough to be held together by gravity,” said SwRI scientist Dr. David Nesvorny, the lead author of the paper “Trans-Neptunian Binaries as Evidence for Planetesimal Formation by the Streaming Instability” published in Nature Astronomy.
During the initial stages of planet growth, dust grains gently collide and chemically stick together to produce larger particles. However, as grains grow larger, collisions likely become more violent and destructive. Scientists have struggled to understand how planetary growth passes the “meter-size barrier.“
The streaming instability theory posits that as large dust grains interact with the gas that orbits young stars, streaming mechanisms cause grains to clump into dense regions and collapse under their own gravity to form planetesimals.
The team studied objects beyond Neptune that orbit each other as binary pairs in the Kuiper Belt. Unlike comets flung past Jupiter or asteroids bombarded by radiation or colliding into each other, the distant Kuiper Belt has not been disturbed much since it formed, so these primordial objects provide hints about the early solar system. If a pair orbits in the same direction as the planets, it’s considered heads-up. It’s tails-up if it orbits in the opposite direction.
Using the Hubble Space Telescope and the Keck Observatory in Hawaii, the team found that most binaries, about 80%, orbit heads-up, which astronomers call “prograde.” This finding contradicted the theory that binaries form when two passing planetesimals are captured into a binary. That theory predicts mostly tails-up or “retrograde” orbits.
To test whether the streaming instability could explain these Kuiper Belt binaries, the team analyzed simulations on large supercomputers. They found that the dense clumps formed by the streaming instability rotated heads-up 80% of the time, in agreement with Kuiper Belt objects.
“While our simulations can’t yet follow the collapse all the way to forming binaries, it appears we are on the right track,” said SwRI’s Dr. Jacob B. Simon, who coauthored the paper.
“The solar system offers many clues to how planets formed, both around our Sun and distant stars,” Nesvorny said. “Although, these clues can be difficult to interpret, observers and theorists working together are starting to make heads or tails of these clues — and the evidence is mostly heads.”
SwRI Wins Contract for Military Support
The U.S. Department of Defense awarded an SwRI-led team a contract to prototype a system to process, exploit and disseminate data from satellites for tactical use by military units. SwRI is collaborating with commercial entities to support the Space and Missile Systems Center CASINO PED ground station initiative.
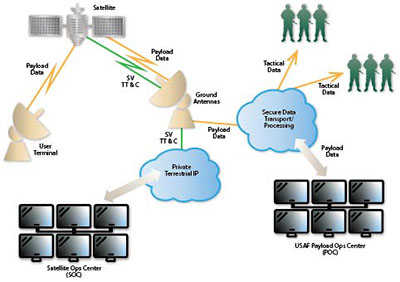
The Commercially Augmented Space Inter-Network Operations (CASINO) Processing, Exploitation, and Dissemination (PED) program seeks to produce and relay more timely combat information and actionable intelligence to the field. Over the past decade, the military has significantly increased the intelligence, surveillance and reconnaissance (ISR) data it collects. Unfortunately, this data surplus has created a processing and analysis bottleneck. The DoD’s Defense Innovation Unit (DIU) will facilitate this technology demonstration and support the tempo needed to address future tactical threats.
“This prototype will demonstrate rapid delivery of processed satellite data to the tactical units needing the information in areas of conflict,” said Debi Rose, SwRI senior program manager leading the effort. “Through this demonstration project, we will integrate a ground network supporting a constellation of satellites with unique processing capabilities and cloud access to deliver data needed to meet military objectives.”
As the systems engineering lead, SwRI will integrate commercial technology including data networks, processing capabilities and delivery. The system will acquire raw data from the satellites and transport it through a commercial gateway to cloud-based services. The data will be processed en route to support rapid delivery of tactical products to in-theater users with minimal human-machine interface.
Active-Vision Automates Traffic Monitoring
SwRI has released Active-Vision™, a machine vision tool that transportation agencies can use to autonomously detect and report traffic condition changes. Active-Vision’s algorithms process camera data to provide real-time information on weather conditions and other issues affecting congestion. Designed for integration with intelligent transportation systems (ITS), ActiveVision can be configured with existing traffic cameras to analyze roadway conditions with no human monitoring required.
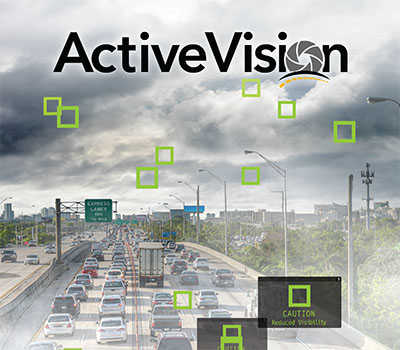
“The goal is to help transportation officials enhance their ITS capabilities with advanced algorithms that autonomously scan vast amounts of visual data, extracting and reporting actionable data,” said Dan Rossiter, an SwRI senior research analyst leading ActiveVision development.
Active-Vision integrates with the SwRI-developed ActiveITS software as well as other ITS and advanced traffic management systems used by state and local agencies across the country. A leader in transportation and traffic management software, SwRI has over 20 years of experience developing and deploying ITS software for state and local agencies. SwRI-developed intelligent transportation systems have been applied to more than 13,000 miles of urban and rural managed roadways in 10 states and Puerto Rico.
“Work in the ITS arena inspired our team to find a solution that could be integrated agnostically into just about any advanced traffic management system using existing cameras and infrastructure,” added Dr. Steve Dellenback, vice president of SwRI’s Intelligent Systems Division.
Modeling material failures to improve armor
SwRI is one of three organizations to win $127,000 in funding at the Ground Vehicle Materials Flash-to-Bang (GVM F2B) Pitch Day. Dr. Alexander Carpenter, Dr. Sidney Chocron and Dr. James Walker of SwRI’s Mechanical Engineering Division proposed developing a computer model to ultimately help make armored vehicles stronger and less susceptible to failure and cracking in combat situations.
The GVM F2B Pitch Day is an innovative procurement approach to jump-start ideas in the accelerating technology ecosystem. Organizations pitched science and engineering proposals focused on significantly lightening or considerably improving ground vehicles or support systems to a panel of U.S. Army officials.
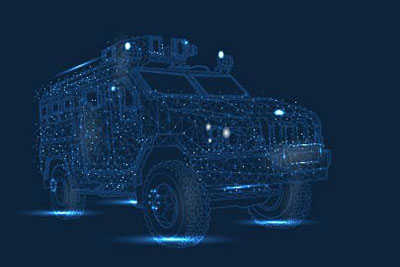
“Normally, armored vehicles are covered in these thick pieces of aluminum that eventually fail as a result of an impact,” Chocron said. “We want to know how that failure starts and propagates. Current computer analyses cannot tell us that.”
In a combat zone, armored vehicles are subjected to ballistic impacts, mine blasts and gunfire. The military relies on computational modeling as well as active testing of the armor on vehicles. However, the Army cannot currently predict the severity or extent of failure in the armor.
“We believe we can do a lot better,” Carpenter said. “By properly modeling the onset and growth of failure, we can increase the accuracy and efficiency of the computational model, making the entire evaluation process more cost-effective because fewer tests are needed.”
The team will create their computational model over the next six months in collaboration with the Army Futures Command and the Ground Vehicle Systems Center.
“Our goal is to improve the modeling the Army does every day for these types of systems,” Carpenter said. “We’re making it more cost-effective and user-friendly with the ultimate goal of helping create tougher, safer vehicles for our soldiers.”
SWRI Designing novel pilot plant for DOE
SwRI is designing a large-scale flameless pressurized oxy-fuel combustion pilot plant for the U.S. Department of Energy. Nearly $3 million in DOE funding will be augmented by $760,658 from industry collaborators to fund the demonstration plant, which will be located near the University of Wyoming.
“This will be a completely new type of power plant,” said Joshua Schmitt, the SwRI project lead. “Oxy-fuel combustion plants generally don’t exist at this scale because the technology is so new.”
The process uses 95% pure oxygen combined with a fuel, typically coal or natural gas. The mixture is fed into a stream of carbon dioxide (CO2) and water inside a combustor. The fuel and oxygen chemically react, producing hot gas that heats the water into steam, which drives a turbine and generates power. Pressurized oxy-fuel combustion has the potential to actuate a turbine directly, potentially improving the overall cycle efficiency.
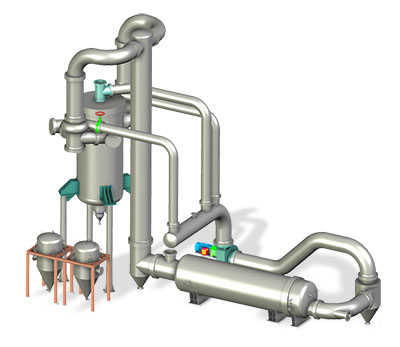
The SwRI-developed oxy-fuel pilot plant includes a vertically designed combustor. The gas and slurry are injected from the top, and the temperature and velocity are tuned using computational fluid dynamics.
Oxygen-rich combustion emits nearly pure CO2 that can be captured and stored or reused rather than released into the atmosphere as a pollutant.
The proposed pilot plant will use coal, which also produces incombustible ash. A novel combustor design mechanically incorporates the ash into small, manageable pellets for disposal.
“One of the most exciting aspects of oxy-fuel combustion is its potential for power generation with almost no CO2 emissions in the atmosphere,” Schmitt said. “Burning coal is often associated with harmful emissions, but this is a way to utilize it for power generation with very limited emissions.”
Over the next year, Schmitt and his collaborators will produce a front-end engineering design study to detail the basic engineering, full design, cost analysis and schedule metrics for building the large-scale pilot plant.
Aquifer Memoir
Four SwRI scientists lent their groundwater management expertise to a new book about the Edwards Aquifer, the primary water source for the San Antonio area. The 27-chapter memoir, titled “The Edwards Aquifer: The Past, Present and Future of a Vital Water Resource,” compiles research spanning decades and addresses emerging challenges facing the aquifer.
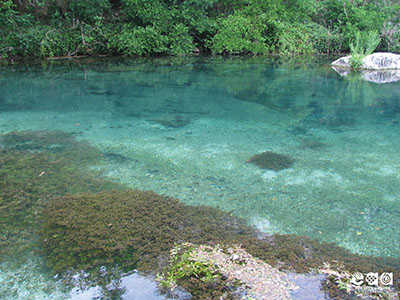
“This first-of-its-kind, comprehensive memoir pulls together all of the information we have about the Edwards Aquifer to date in one place,” said Dr. Ronald Green, an Institute scientist and groundwater hydrologist who co-edited the book and coauthored several chapters. “Researchers, regulators and water authorities can use this memoir to manage the aquifer and build upon the work that’s already been done.”
The book covers a range of topics, characterizing the aquifer’s components and hydrogeologic structure and how climate and urbanization affect the system. Green says thoroughly understanding the demands on the aquifer, which provides water to millions of people, is key to preserving the resource for future Texans.
“Over time, the aquifer has been used and developed more,” Green said. “Understanding the limitations of the aquifer will help guide its management to protect the availability and quality of the water. This book can provide policymakers with a roadmap of where to develop and at what levels.”
All contributors are recognized experts on the Edwards Aquifer, including SwRI scientists Dr. David Ferrill, Dr. Beth Fratesi and Rebecca Nunu, who coauthored chapters of the book.
Published by the Geological Society of America (GSA), the memoir is currently available in print and digital versions. Proceeds from the sale of the book will go to GSA, a nonprofit, international scientific society that supports Earth science education.
Saturn’s Rings
No one knows for certain when Saturn’s iconic rings formed, but a new study cotauthored by an SwRI scientist suggests that they are much older than some scientists think.
The study takes a closer look at 2017 Cassini spacecraft data that inspired several research papers suggesting that the rings were formed around the time dinosaurs roamed the Earth. Those studies, published in 2018 and 2019, challenged long-held models that put the formation of the rings several billion years earlier, around the time Saturn formed with the rest of the solar system.
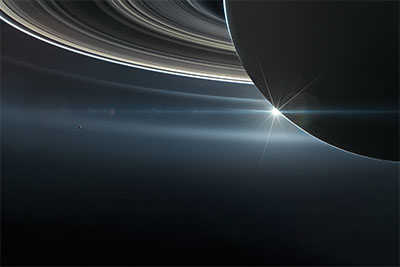
Courtesy of NASA/JPL-Caltech
In the latest twist, SwRI scientist Luke Dones and his collaborators argue that the historic models probably had it right in the first place. The age debate centers around a trove of Cassini data that revealed dazzling images of Saturn’s rings composed of clear, almost pure water ice.
“After Cassini’s mission ended, some research claimed the rings must be younger than we had considered them to be. Scientists argued that if the rings were much older, they would have become much more polluted as a result of meteoroids crashing into them,” Dones said.
The studies suggested that the rings would have absorbed portions of dark, dusty material from the meteoroids and gradually dimmed. Therefore, the rings appeared too bright and clean to have existed in the solar system for billions of years.
Dones and his collaborators pointed out that Cassini measurements also show the rings are constantly losing matter to Saturn. The mysterious process could very well be “cleaning” the icy rings and making them brighter over time. One of the clearest indications that the rings are old is that their mass is consistent with researchers’ current understanding of how primordial rings change.
“It’s not impossible to determine the age of the rings, but to do so we’ll need a future mission to Saturn to study the rings themselves as well as the relationship between them and the gas giant,” Dones said.
Hypersonic Flight
To advance hypersonic flight research, SwRI conducted a series of sub-scale tests to elucidate the conditions future aircraft may experience traveling faster than 10 times the speed of sound.
“Hypersonic speed is defined as faster than five times the speed of sound or greater than Mach 5. When something is flying that fast, the air will chemically decompose around the craft,” said SwRI’s Dr. Nicholas J. Mueschke, who led the internally funded research. “Some points behind the shockwave created by the vehicle are hotter than the surface of the sun. Essentially, it’s flying through this strange chemical environment that causes whatever is traveling through it to heat up, melt and chemically react with the air.”
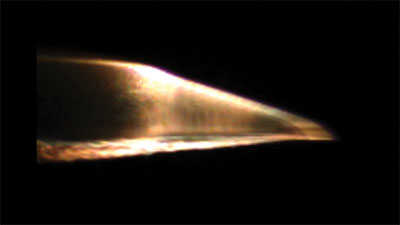
SwRI engineers used high-speed video to image a conical flight body launched from our two-stage light gas gun at Mach 14.8 or 11,400 mph. Material stripped off the flying object is so hot that it glows, allowing the object to be photographed in flight.
Because that environment is so unique, recreating realistic flight conditions to test vehicles for hypersonic flight is a challenge. Wind tunnels can match some of the conditions, but don’t fully replicate the pristine atmospheric and chemical reactions that a hypersonic vehicle experiences in flight. Mueschke and his colleagues used SwRI’s two-stage light gas gun system to reproduce realistic hypersonic flight conditions.
The 72-foot gun system is designed to generate very high velocities — up to15,660 miles per hour — and is typically used to study ballistics. SwRI engineers used the system to propel sub-scale objects at speeds from Mach 10 to 15 to study how hypersonic flight conditions affect a variety of materials and geometries.
The facility mimics a broad range of flight altitudes in an acoustically and chemically pristine environment. The flights of these smaller projectiles replicate the true hypersonic flight conditions full-scale vehicles will experience. The research simulates the intense heating and vehicle material loss hypersonic vehicles will experience due to turbulent boundary layer transitions and complex shock wave interactions.
“This research will help us address material problems associated with hypersonic flight, the first step toward the technology of tomorrow,” Mueschke said.
New Tribology Test Rig
SwRI recently added an additional single cam rig (SCR) to its considerable array of tribology test equipment. The rig investigates wear mechanisms in engine valvetrain components.
Tribology is the study of interacting surfaces in relative motion, focusing heavily on friction, wear and lubrication. Though it’s most often applied to the automotive industry, SwRI has used tribology to solve problems in areas ranging from aerospace to cosmetics to manufacturing.
“We don’t just answer a question,” said Dr. Peter Lee, who led the SCR development team. “We offer the entire package. We address the problem, provide answers and find a solution. If there isn’t a device to help us do that, we create it ourselves.”
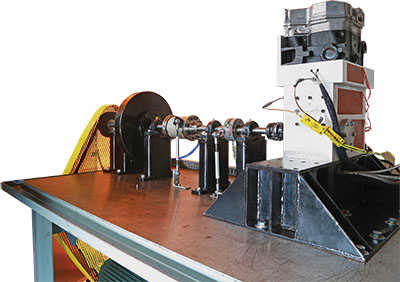
SwRI designed this single cam rig to investigate wear mechanisms in an engine’s valvetrain components.
Lee and his group created the first SCR in 2014 to make the ASTM D7484 wear test for engine oil more efficient and cost-effective. The custom-designed SCRs serve as platforms for detailed lubricant research. Using actual engine components in the rig reduces costs while providing more detailed understanding of wear mechanisms over standard tribology equipment.
“In the years since we created the first single cam rig, the demand for tests has become so high that it became necessary for us to build a second one,” Lee said.
Both rigs have the unique capability to measure a lubricant’s effect on the rotation of the lifter, a vital engine component that actuates the intake and exhaust valves, without interfering with the natural motion of the other valvetrain components. The tests help to understand properties such as wear and film formation. Computer control allows the replication of any engine test cycle.
“The fun of tribology is that it enables us to tackle so many unique challenges with a great deal of ingenuity and creativity,” Lee said. “Tribology feeds into practically every industry there is.”
2D Radar Retroreflector Measures Subtle Ground Movements
An SwRI team designed a two-dimensional radar retroreflector that remotely monitors subtle shifts in the Earth’s crust. The patent-pending Van Atta retroreflector works in conjunction with satellites to precisely measure ground movement caused by earthquakes, oil production, mining and other processes. Movement can pose a risk to critical infrastructure such as nuclear facilities, airports and bridges.
“By monitoring shifts in the Earth’s crust, emergency managers, city leaders or anyone with an interest in community safety can detect and anticipate instabilities in a particular area,” said SwRI Senior Research Scientist Dr. Marius Necsoiu, who created the Van Atta retroreflector concept with support from SwRI engineers Emilio Martinez and B. David Moore.
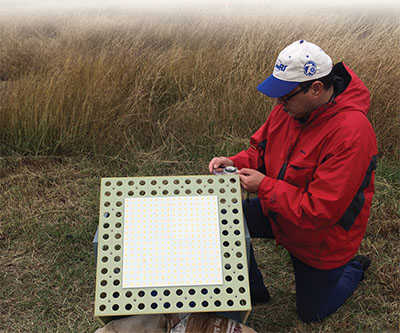
Dr. Marius Necsoiu sets up a Van Atta retroreflector for testing and method validation. Retroreflector panels measure less than 1-by-1 foot and can be painted to match any surface.
The device incorporates an antenna array patented by Dr. L.C. Van Atta in 1959 that reflects energy over a wide range of angles. SwRI’s retroreflector merges Van Atta’s principles with radar interferometry, a satellite-based method of measuring ground movement with radar signals. When monitored over time, the reflected signals show whether the ground in a particular location is shifting, detecting even slight movement.
“Analyzing subtle changes from space requires markers on the ground that don’t change over time,” Necsoiu said. “The compact Van Atta retroreflector provides that consistency. The flat design allows secure, flush mounting to structures or the ground. In addition, the retroreflector can withstand a range of challenging environments and temperatures, making it ideal for this type of data collection.”
Traditional 3D reflectors are bulky and susceptible to damage or vandalism. SwRI’s 1-by-1-foot panels can be painted to match any surface and configured in various patterns, making them easier to conceal and less prone to damage.
SwRI’s Van Atta retroreflector can be adapted for a range of frequencies, making it compatible with any satellite, drone or radio device. This technology is currently available for government and industry projects.
Algorithm Aids Search for Exoplanets
Inspired by movie streaming services such as Netflix and Hulu, an SwRI scientist has developed a technique to look for stars likely to host giant, Jupiter-sized planets outside of our solar system. She developed an algorithm to identify stars likely to have these exoplanets based on the composition of stars known to have planets.
“My viewing habits have trained Netflix to recommend sci-fi movies I might like — based on what I’ve already watched. These ‘watched movies’ are like known star-exoplanet systems,” said Dr. Natalie Hinkel, a planetary astrophysicist at SwRI. “Then, the algorithm looks for stars with yet-undetected planets — which are comparable to movies I haven’t watched — and predicts the likelihood that those stars have planets.”
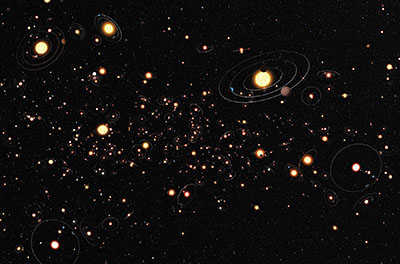
Courtesy of ESO/M. Kornmesser
This artist’s impression (not to scale) illustrates how common star-exoplanet systems may be in the Milky Way. An SwRI scientist has developed an algorithm to predict the likelihood that a star hosts giant planets, based on the composition of known star-exoplanet systems.
Hinkel used the Hypatia Catalog (hypatiacatalog.com), a publicly available stellar database she developed, to train and test the algorithm. It’s the largest database of stars and their elements for the population within 500 light years of our Sun. At last count, Hypatia had stellar element data for 6,193 stars, 401 of which are known to host planets. The database also catalogs 73 stellar elements from hydrogen to lead.
Hinkel’s team identified around 360 potential giant planet host stars that have more than a 90% probability of hosting a giant exoplanet. “We were excited, so we used archival telescope data to search for any signs of planets around these likely host stars,” Hinkel said. “We identified possible Jupiter-sized planets around three stars predicted by the algorithm.”
When asked about how reliable her algorithm is, she explained that “We don’t have any true-negatives in our data — that is, stars that we know don’t have planets — so we ‘hid’ some known planet-hosting stars in the data to see what their prediction score would be like. On average they scored more than 75%, which is great! That’s probably a higher average than me liking the sci-fi movies Netflix picks for me.”