Background
Battery usage is expected to grow dramatically in upcoming years across various sectors. Lithium-ion (Li-ion) batteries are among the most used energy storage technologies due to their high energy densities and specific energy capacities. These desirable characteristics also make this technology a potential safety hazard. When exposed to certain abuse factors, these batteries experience thermal runaway that can lead to fire and particulate/gaseous emissions. In the real-world, these events can be initiated by exposure to abuse factors such as thermal (over-heating), electrical (over-charging) or mechanical (physical). Detailed understanding of emissions from such fires is important to assess impact on human health and environment.
Approach
This program was focused on performing detailed characterization of particle emissions, and limited characterization of gaseous emissions from Li-ion battery systems experiencing thermal runaway. Experiments were conducted to understand the level of variability of emissions, influence of initiation (or abuse) mechanism, and impact of battery chemistry on particle and gaseous emissions. Four identical lithium iron phosphate (LFP) modules and one nickel manganese cobalt oxide (NMC) module were each subjected to thermal runaway. Two LFP modules and the NMC module were triggered into runaway via nail-penetration, while the remaining two LFP modules were triggered into runaway via over-charging. Particle emissions were characterized in terms of particulate matter mass (PM2.5), real-time total (solid + volatile) and solid PN/size (5.6- 560 nm), real-time black carbon, organic/elemental carbon partitioning and volatile fraction of PM2.5. Gases were measured using a Fourier Transform Infrared spectrometer.
Accomplishments
Results suggest that battery fires can result in significant particle and gaseous emissions that may be a function of initiation mechanism, battery chemistry, and cell arrangement within a module among other variables. LFP modules subjected to nail penetration yielded less emissions compared to all other cases as thermal runaway didn’t propagate to all cells within the module. Over-charging LFP modules resulted in significant emissions that showed a continuous increase till peak levels were reached after which a gradual decrease was observed (Figure 1). PM2.5 emissions of the order of 380 g/hour and total PN emissions of the order of 1.5E+17 particles/hour were observed. Nail-penetration of the NMC module resulted in several distinct peaks due to propagation of runaway from cell to cell (Figure 1). This module resulted in the highest emission rates of 551 grams/hour of PM2.5 and 2.1E+17 particles/hour of total PN. For all the above cases, peak emission levels were found to be in the ultrafine (sub-100 nm) size range, well within the respirable size range of the human respiratory system. Battery chemistry also influences the nature of hazardous gaseous emissions. The LFP overcharge tests yielded hydrofluoric acid that exceeded immediately dangerous to life and health (IDLH) limits (30 ppm) while the NMC nail penetration test yielded formaldehyde beyond IDLH limits (20 ppm). Results from this study highlight how significant emissions can be when batteries enter thermal runaway. This demonstrates the need for establishing a regulatory framework focused on understanding and mitigating such emissions from different battery technologies.
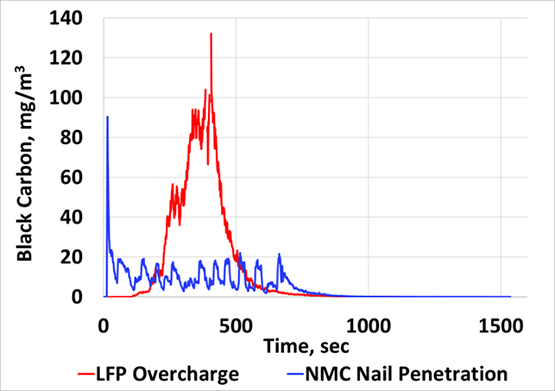
Figure 1: Real-time black carbon emissions profile for LFP overcharge and NMC nail penetration test.